MILLIMETER WAVES
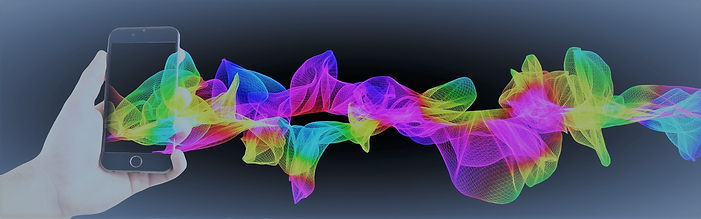
Today's wireless networks face a significant challenge: the increasing number of users and devices is consuming more data than ever, yet this data is confined to the same radio-frequency spectrum bands that mobile providers have historically utilised. This congestion results in reduced bandwidth for everyone, leading to slower services and more frequent dropped connections.
​
To address this issue, one solution has been to utilise a completely new segment of the spectrum previously untapped for mobile services. This has led to the adoption of millimetre waves in broadcasting, which operate at higher frequencies than the traditional radio waves used for mobile phones.
​
Millimetre waves operate within the 30 to 300 gigahertz range, unlike the sub-6 GHz bands that were traditionally employed for mobile communications. Their name derives from their wavelength, which ranges from 1 to 10 mm, in contrast to the longer radio waves, measuring tens of centimetres, that support current smartphones.
​
Previously, millimetre waves were primarily used by satellite and radar systems for specific applications. However, with the advent of 5G, cellular providers have started to utilise them for point-to-point data transmission, such as between base stations. Extending their use to connect mobile users with nearby base stations represents a novel application of millimetre wave technology.
​
Nevertheless, millimetre waves come with a significant limitation: they struggle to penetrate buildings or obstacles and can be absorbed by foliage and rain. To overcome this, 5G networks are incorporating another innovation: small cells.
SMALL CELLS
SMALL CELLS

Small cells are portable miniature base stations that require minimal power to operate and can be placed approximately every 250 meters throughout cities. To ensure continuous connectivity, carriers could install thousands of these stations in a city to form a dense network that acts like a relay team, receiving signals from other base stations and sending data to users at any location.
While traditional cell networks have also come to rely on an increasing number of base stations, the transition to 5G has necessitated an even more extensive infrastructure. However, the antennas used for small cells can be significantly smaller than those in traditional setups, especially when they are designed to transmit the tiny millimetre waves. This reduction in size facilitates the installation of small cells on items like light poles and building tops, integrating them smoothly into the urban environment.
​
This innovative network structure offers a more precise and efficient spectrum usage. The increase in station density means that frequencies utilised by one station in a specific area can be reused by another station in a different location, effectively serving another user without interference. However, the vast number of small cells required for a comprehensive 5G network presents a challenge, particularly in extending this level of coverage to rural areas.
​
Moreover, 5G base stations are equipped with a significantly higher number of antennas compared to those in the 4G era, capitalising on the advancements brought by another pioneering technology: massive MIMO.
MASSIVE MIMO
4G base stations typically feature around a dozen antenna ports that manage cellular traffic, with eight dedicated to transmitters and four for receivers. In contrast, 5G base stations have evolved to support approximately a hundred ports, allowing for a significant increase in the number of antennas on a single array.
This advancement enables a base station to simultaneously communicate with many more users, potentially augmenting the capacity of mobile networks by more than 22 times.

This development is largely due to the technology known as massive MIMO, which stands for multiple-input multiple-output. MIMO technology, which is already present in some 4G base stations, utilises at least two transmitters and receivers to simultaneously send and receive greater amounts of data. Massive MIMO amplifies this principle by incorporating dozens of antennas on a single array, taking data transmission capabilities to unprecedented levels.
While MIMO technology is a component of some 4G base stations, massive MIMO has transitioned from being an experimental technology, previously confined to labs and limited field trials, to a critical element of deployed 5G networks. In its initial tests, massive MIMO set new benchmarks for spectrum efficiency, a key metric that quantifies the volume of data that can be transmitted to a specific number of users per second.
Massive MIMO is now a cornerstone in the development of 5G, contributing significantly to its enhanced capacity and efficiency. However, the introduction of many more antennas to manage cellular traffic has also raised the challenge of increased signal interference. That’s why 5G stations must incorporate beamforming.
BEAMFORMING
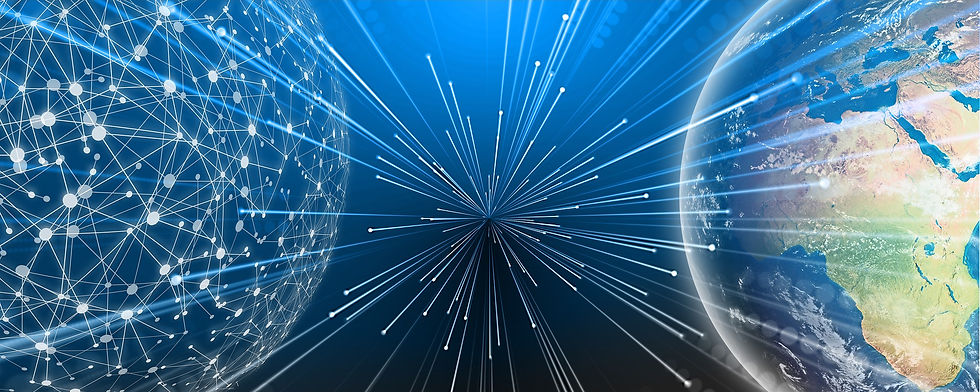
Beamforming is a traffic-signaling system for cellular base stations that identifies the most efficient data-delivery route to a particular user, and it reduces interference for nearby users in the process. Depending on the situation and the technology, there are several ways for 5G networks to implement it.
​
Beamforming can help massive MIMO arrays make more efficient use of the spectrum around them. The primary challenge for massive MIMO is to reduce interference while transmitting more information from many more antennas at once. At massive MIMO base stations, signal-processing algorithms plot the best transmission route through the air to each user. Then they can send individual data packets in many different directions, bouncing them off buildings and other objects in a precisely coordinated pattern. By choreographing the packets’ movements and arrival time, beamforming allows many users and antennas on a massive MIMO array to exchange much more information at once.
​
For millimeter waves, beamforming is primarily used to address a different set of problems: Cellular signals are easily blocked by objects and tend to weaken over long distances. In this case, beamforming can help by focusing a signal in a concentrated beam that points only in the direction of a user, rather than broadcasting in many directions at once. This approach can strengthen the signal’s chances of arriving intact and reduce interference for everyone else.
​
Besides boosting data rates by broadcasting over millimeter waves and beefing up spectrum efficiency with massive MIMO, wireless engineers are also trying to achieve the high throughput and low latency required for 5G through a technology called full duplex, which modifies the way antennas deliver and receive data.
FULL DUPLEX
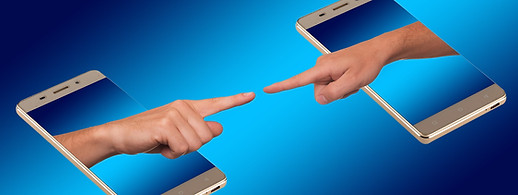
Some militaries already use full duplex technology that relies on bulky equipment. To achieve full duplex in personal devices, researchers must design a circuit that can route incoming and outgoing signals, so they don’t collide while an antenna is transmitting and receiving data at the same time.
​
This is especially hard because of the tendency of radio waves to travel both forward and backward on the same frequency—a principle known as reciprocity. But recently, experts have assembled silicon transistors that act like high-speed switches to halt the backward roll of these waves, enabling them to transmit and receive signals on the same frequency at once.
​
One drawback to full duplex is that it also creates more signal interference, through a pesky echo. When a transmitter emits a signal, that signal is much closer to the device’s antenna and therefore more powerful than any signal it receives. Expecting an antenna to both speak and listen at the same time is possible only with special echo-canceling technology.